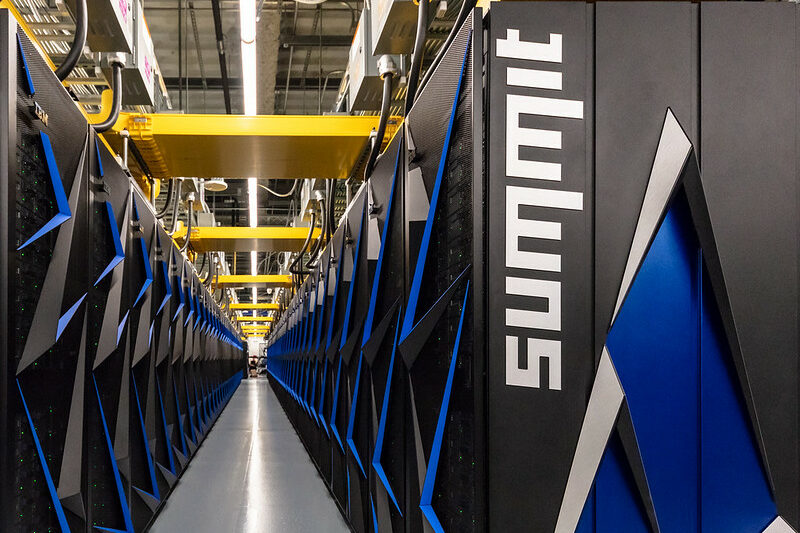
2021
Supercomputing support
Benjamin List, whose research was supported by modeling and simulation efforts on supercomputers at ORNL, shared the 2021 Nobel Prize in Chemistry.
ORNL supercomputers support Nobel Prize-winning research
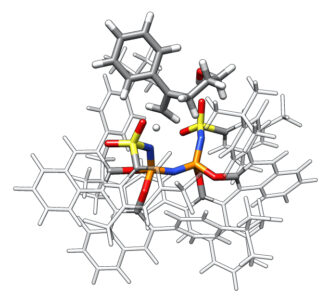
The asymmetric core of the catalyst molecule (colorful atoms in the center) and a molecule sitting on top of the catalyst (grey). Image Credit: Dmytro Bykov, ORNL
A scientist whose research was supported by modeling and simulation efforts on supercomputers at ORNL shared the Nobel Prize in Chemistry 2021.
The pair who won the prize, Benjamin List of the Max-Planck-Institut für Kohlenforschung and David MacMillan of Princeton University, developed a new and highly selective way of constructing chiral molecules, molecules which are mirror reflections of each other. This was achieved through asymmetric organocatalysis, a process in which an organic molecule serves as a catalyst that drives a chemical transformation to one desired product.
Typically, synthesizing catalysts is tedious experimental work. It requires careful planning and a working hypothesis of the reaction mechanism to fine-tune the catalyst’s activity. However, the process is much more complex in real life than it is on paper because the details of the reaction mechanism are not known.
The team led by List at the Max-Planck-Institut required computer modeling of the phenomenon to augment their physical experiments running at the lab. That’s where the Oak Ridge Leadership Computing Facility (OLCF) and Dmytro Bykov, a computational scientist at ORNL, came in. Bykov used the 200-petaflop Summit, the OLCF’s flagship supercomputer, to simulate the team’s new catalysts, turning molecules into specific end products. The modeling helped the team determine whether the new catalysts would be effective.
The team specifically studied the role of catalysts in the conversion of inert molecules called olefins. Olefins are among nature’s most abundant chemical compounds and are commonly obtained from crude oil. They are of interest in biochemistry because they are ideal for use as precursors to drug compounds and other chemicals.
Catalysts work by lowering the energetic barriers of a chemical transformation and thus directing a molecule toward particular products. However, the products can be chiral, meaning they share the same composition and a similar structure but are flipped left or right from each other. Catalysts can force molecules into a left or right conformation, and these different positionings can have completely different effects — for example, in a human body.
“In a pairing, the left molecule might be a drug, and the right molecule might even be poison in some extreme examples,” Bykov said. “For this reason, it’s important to synthesize these into a specific direction.”
Without good catalysts, scientists might end up with a mixture of left and right molecules that could have deleterious effects. Organocatalysis offers a way around this problem.
“The team had theorized the most promising catalysis candidates, but they needed details on the reaction mechanisms that are difficult to determine in a lab setting,” Bykov said. “For instance, computer modeling can find structures of transition states, reaction barriers, and alternative pathways and ultimately shed light on the nature of substrate activation.”
Bykov’s simulations gave the team a way of determining how to stress a molecule in a specific way to move it closer to the desired product.
“In an experiment, the initial hypothesis may not work, and you may get something that you think should have reactivity, but it doesn’t,” Bykov said. “Computational modeling may help direct experimental efforts so that we can synthesize these new and important organic catalysts in more predictive and rational way.” —Rachel McDowell